Use of 3D-printed model to plan the surgical management of a patient with isolated orbital floor fracture: a case report
Highlight box
Key findings
• A three-dimensional (3D) printed model was successfully used to plan the surgical treatment of a patient with an orbital floor fracture, as well as to pre-cut a Codubix prosthesis, which was subsequently used to reconstruct the fractured bone.
What is known and what is new?
• Orbital floor fractures typically manifest as eyeball mobility disorders with double vision (diplopia), enophthalmia, and infraorbital paresis. Surgical treatment of these fractures involves orbital floor reconstruction, which requires great precision.
• 3D printing seems to be a useful method that allows more thorough preparation for the surgery and also could potentially shorten its duration.
What is the implication, and what should change now?
• 3D-printed models can be prepared based on pre-operative computed tomography (CT) scans and used to thoroughly prepare for the surgery in patients with orbital floor fractures.
Introduction
Physical assault and traffic accidents has been reported to be the most common cause of orbital fractures (1,2). Two theories have been put forward to explain the pathomechanism of orbital floor fractures. The first theory postulates that blunt trauma to the soft tissues of the orbit results in increased orbital pressure and causes fracture of the floor. According to the second theory, the walls of the orbit bend and then break in response to the posterior movement of the infraorbital rim (3,4).
An orbital floor fracture is typically manifested as eyeball mobility disorders with double vision (diplopia), enophthalmia, and hypoesthesia in the area innervated by the infraorbital nerve (3).
The indications for surgical treatment of orbital floor fractures remain controversial (5,6). Traditionally, these include persistent diplopia and severe enophthalmia. The procedure involves freeing the trapped tissues from the lumen of the maxillary sinus and rebuilding the orbital floor (7,8). It is assumed that non-resorbable materials or autogenous bone should be used in extensive fractures, while resorbable materials would find usage in smaller fractures. The choice of material however remains a topic of ongoing debate (9).
Technological progress in three-dimensional (3D) printing allows physical prototyping of implants. Creating a model that faithfully reproduces a given patient’s anatomical conditions facilitates accurate planning of the surgical procedure (10). The requisite for obtaining faithful 3D models is high-quality image acquisition. We present this case in accordance with the CARE reporting checklist (available at https://acr.amegroups.com/article/view/10.21037/acr-24-73/rc).
Case presentation
A 43-year-old female patient presented to the hospital with diplopia, which first occurred immediately after she fell from her own height. Her medical history included arterial hypertension, without any additional accompanying disease. Physical examination revealed edema of the left infraorbital region, left-sided infraorbital paresis, and diplopia while looking up or down. The pupils were wide, symmetrical, and reacted correctly to light.
Computed tomography (CT) revealed a fracture of the floor of the left orbit. The bone was fragmented, and the bony fragments were displaced into the maxillary sinus. The fracture involved the infraorbital canal. Displacement of the inferior rectus and intraorbital fat tissue into the maxillary sinus was observed. Air bubbles were visible in the soft tissues surrounding the orbit (Figure 1).
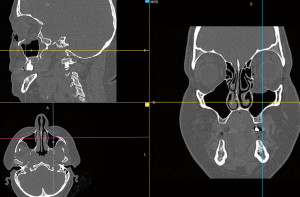
The patient qualified for surgical treatment. Due to the size and type of tissue damage, a stereolithographic anatomical model was prepared using 3D printing. The patient underwent a CT scan of the orbits with layers 0.75 mm in size using a standard soft tissue filter (512×512 pixels matrix). The data were saved in a standard Digital Imaging and Communications in Medicine (DICOM) file. A high signal-to-noise ratio (SNR), adequate soft tissue contrast, and good spatial resolution with thinner voxels (0.25 to 1.25 mm) allowed the gathering of required information for 3D reconstruction of the orbital floor.
Using the open-source software “InVesalius” (3.1.1, Brazil), segmentation based on a threshold in grey scale was performed and a region of interest (ROI) was selected, which allowed the generation of a 3D volumetric reconstruction of the skull (Figure 2).
The data prepared in this manner were exported in the form of a stereolithography (STL) file. The correctness of the generated 3D model was checked using the “Autodesk Meshmixer” program (11.0.544). The final data were sent to the printer using the “Pre-Form” program (1.2.9, Formlabs). To produce the model, a low-force stereolithography system (LFS) (Form 3, Formlabs) and BioMed Clear resin (ISO 13485) were used. The printing temperature was set to 210 ℃. The layer thickness was set at 50 µm. The final models were sterilized in an autoclave and used to prepare the prosthesis during surgery.
A polypropylene prosthesis “Codubix Oczodół” (Tricomed, Poland) was used in the surgical treatment. The procedure was performed on the 5th day after trauma under general anesthesia using the transconjunctival approach. The prosthesis was intraoperatively adapted and pre-cut ex vivo using the 3D-printed model of the fractured orbit first, and then inserted into the orbit to reconstruct the orbital floor (Figures 3-7).
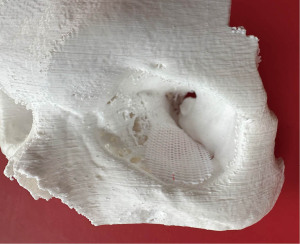
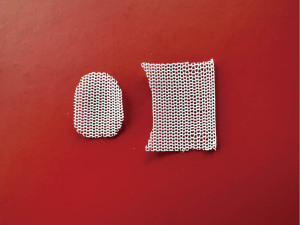
No complications were observed during the postoperative course. Instant improvement in diplopia was noted. A CT scan was performed on the 3rd day after surgery. No herniation into the maxillary sinus was observed (Figure 8).
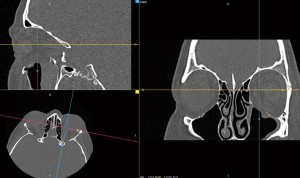
All procedures performed in this study were in accordance with the ethical standards of the institutional and/or national research committee(s) and with the Helsinki Declaration (as revised in 2013). Written informed consent for publication of this case report and accompanying images was not obtained from the patient or the relatives after all possible attempts were made.
Discussion
It is a well-known fact that proper manual training and thorough anatomical knowledge are needed to perform surgical procedures efficiently and appropriately. However, even the most detailed anatomical education does not include the individual characteristics of the operated patient. Therefore, surgeons have always been interested in methods that allow more thorough and individualized surgery planning. This possibility is partially supported by the standard radiological techniques. However, the information acquired via radiology is limited, and it is an arduous task to predict the conditions that could be found inside the patient based solely on them (11). Therefore, numerous ideas for use of modern technologies such as virtual or augmented reality, computer modelling, and 3D printing have been developed.
The first attempts to produce 3D-printed models to resemble human anatomy can be traced back to the 1990s (12). Less than a decade later, attempts to use such models in surgery planning and intraoperative navigation have been undertaken (13,14). It is important to note that these attempts were undertaken inter alia in the field of head and neck surgery (12). Nowadays 3D printing is widely used in medicine for the production of personalized implants as well as in manufacturing precise physical models of patient anatomy, as in this study. Such a model can be used by the surgeon to get acquainted with the individual anatomy of the patient. Moreover, it also offers a unique possibility to touch, which is not possible using virtual or augmented reality. This allows adjustment of the prosthesis used for an ex vivo surgery, which in theory should shorten the duration of the surgery, which is one of the commonly known factors contributing to the risk of complications (15,16). It also seems to be technically easier. In addition, proper adjustment of the prosthesis ex vivo is possible due to better visibility and lack of interference from soft tissues of the patient. Sterility of the procedure is ensured through sterilization of the prosthesis after contact with the 3D-printed models. If the material cannot undergo sterilization due to technical reasons, sterilization or sterile draping of the model itself can be done and the prosthesis can be adjusted in the operating room. In comparison during a standard operative treatment of an orbital floor fracture the fracture itself is assessed preoperatively only basing on CT-images and clinical presentation. The proper assessment of the shape and size of the fracture can therefore commence only intraoperatively. At this point the material used for the reconstruction is repeatedly put into the orbit and adjusted until it reaches the proper form. To assist this procedure, special templates can be used.
A potential disadvantage of the 3D-model assisted method described in this study could be the increased duration of time required for preparation of surgery, which could be problematic in patients with orbital floor fractures as the available literature indicates that early intervention is a positive prognostic factor (17). However, from our experience, we conclude that a skilled person can prepare the data from the CT scans within 2–3 hours. Furthermore, the 3D printing procedure itself can take somewhere between a few to a dozen hours. Therefore, it can be assumed that this technique could be used in the time available for standard preoperative preparations. The method is also relatively cost-efficient, in our experience adding only about 100 USD to the whole treatment process of a single patient.
Conclusions
3D printing seems to be a useful method that allows more thorough preparation for the surgery and also could potentially shorten its duration.
Acknowledgments
Funding: None.
Footnote
Reporting Checklist: The authors have completed the CARE reporting checklist. Available at https://acr.amegroups.com/article/view/10.21037/acr-24-73/rc
Peer Review File: Available at https://acr.amegroups.com/article/view/10.21037/acr-24-73/prf
Conflicts of Interest: All authors have completed the ICMJE uniform disclosure form (available at https://acr.amegroups.com/article/view/10.21037/acr-24-73/coif). The authors have no conflicts of interest to declare.
Ethical Statement: The authors are accountable for all aspects of the work in ensuring that questions related to the accuracy or integrity of any part of the work are appropriately investigated and resolved. All procedures performed in this study were in accordance with the ethical standards of the institutional and/or national research committee(s) and with the Helsinki Declaration (as revised in 2013). Written informed consent for publication of this case report and accompanying images was not obtained from the patient or the relatives after all possible attempts were made.
Open Access Statement: This is an Open Access article distributed in accordance with the Creative Commons Attribution-NonCommercial-NoDerivs 4.0 International License (CC BY-NC-ND 4.0), which permits the non-commercial replication and distribution of the article with the strict proviso that no changes or edits are made and the original work is properly cited (including links to both the formal publication through the relevant DOI and the license). See: https://creativecommons.org/licenses/by-nc-nd/4.0/.
References
- Cruz AA, Eichenberger GC. Epidemiology and management of orbital fractures. Curr Opin Ophthalmol 2004;15:416-21. [Crossref] [PubMed]
- Manolidis S, Weeks BH, Kirby M, et al. Classification and surgical management of orbital fractures: experience with 111 orbital reconstructions. J Craniofac Surg 2002;13:726-37; discussion 738. [Crossref] [PubMed]
- Kryst L. Chirurgia szczękowo-twarzowa podręcznik dla studentów, Wydanie II Unowocześnione. PZWL Warszawa 1993 [Chapter]. Złamanie Oczodołu, Page:267-274.
- Ahmad F, Kirkpatrick NA, Lyne J, et al. Buckling and hydraulic mechanisms in orbital blowout fractures: fact or fiction? J Craniofac Surg 2006;17:438-41. [Crossref] [PubMed]
- Patel S, Shokri T, Ziai K, et al. Controversies and Contemporary Management of Orbital Floor Fractures. Craniomaxillofac Trauma Reconstr 2022;15:237-45. [Crossref] [PubMed]
- Basta MN, Rao V, Roussel LO, et al. Refining Indications for Orbital Floor Fracture Reconstruction: A Risk-Stratification Tool Predicting Symptom Development and Need for Surgery. Plast Reconstr Surg 2021;148:606-15. [Crossref] [PubMed]
- Harris GJ. Orbital blow-out fractures: surgical timing and technique. Eye (Lond) 2006;20:1207-12. [Crossref] [PubMed]
- Young SM, Sundar G, Lim TC, et al. Use of bioresorbable implants for orbital fracture reconstruction. Br J Ophthalmol 2017;101:1080-5. [Crossref] [PubMed]
- Taxis J, Ungerboeck L, Motel C, et al. Thin PDS Foils Represent an Equally Favorable Restorative Material for Orbital Floor Fractures Compared to Titanium Meshes. Tomography 2023;9:1515-25. [Crossref] [PubMed]
- Bernhard B, Illi J, Gloeckler M, et al. Imaging-Based, Patient-Specific Three-Dimensional Printing to Plan, Train, and Guide Cardiovascular Interventions: A Systematic Review and Meta-Analysis. Heart Lung Circ 2022;31:1203-18. [Crossref] [PubMed]
- Taxis J, Ungerboeck L, Gehrking MR, et al. Two-Dimensional Post-Traumatic Measurements of Orbital Floor Blowout Fractures Underestimate Defect Sizes Compared to Three-Dimensional Approaches. Tomography 2023;9:579-88. [Crossref] [PubMed]
- Barker TM, Earwaker WJ, Frost N, et al. Integration of 3-D medical imaging and rapid prototyping to create stereolithographic models. Australas Phys Eng Sci Med 1993;16:79-85. [PubMed]
- Sodian R, Schmauss D, Markert M, et al. Three-dimensional printing creates models for surgical planning of aortic valve replacement after previous coronary bypass grafting. Ann Thorac Surg 2008;85:2105-8. [Crossref] [PubMed]
- Winder J, Bibb R. Medical rapid prototyping technologies: state of the art and current limitations for application in oral and maxillofacial surgery. J Oral Maxillofac Surg 2005;63:1006-15. [Crossref] [PubMed]
- Sigron GR, Rüedi N, Chammartin F, et al. Three-Dimensional Analysis of Isolated Orbital Floor Fractures Pre- and Post-Reconstruction with Standard Titanium Meshes and "Hybrid" Patient-Specific Implants. J Clin Med 2020;9:1579. [Crossref] [PubMed]
- Cheng H, Clymer JW, Po-Han Chen B, et al. Prolonged operative duration is associated with complications: a systematic review and meta-analysis. J Surg Res 2018;229:134-44. [Crossref] [PubMed]
- Damgaard OE, Larsen CG, Felding UA, et al. Surgical Timing of the Orbital "Blowout" Fracture: A Systematic Review and Meta-analysis. Otolaryngol Head Neck Surg 2016;155:387-90. [Crossref] [PubMed]
Cite this article as: Stopa L, Papeczyc A, Stopa Z, Abed K. Use of 3D-printed model to plan the surgical management of a patient with isolated orbital floor fracture: a case report. AME Case Rep 2024;8:110.